Structured aggregates of bacteria known as biofilms are often able to survive under harsher environmental conditions than their free-living counterparts. One common problem resulting from biofilm hardiness is increased pathogenicity and drug-resistance. From common medical ailments such as urinary tract infections and cavities to life-threatening diseases such as cystic fibrosis and endocarditis, biofilms are often resistant to even the most potent antibiotics.
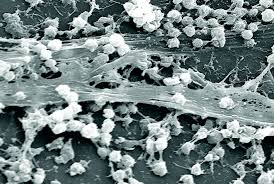
Electron micrograph image of a biofilm at 21, 850x magnification. Biofilms typically consist of structured communities of bacteria and an extracellular polysaccharide matrix that promotes surface adhesion.
The hardiness and pathogenicity of biofilms poses additional risk for humans in one particularly extreme environment: space. On the Mir space station that operated in low Earth orbit until 2001, biofilms were abundant and tenacious, interfering with basic shipboard functions such as water purification. Furthermore, the little research to date suggests bacteria that grow in “microgravity”, the extremely low gravity conditions present in and on the surface of spacecrafts, can be more virulent than their Earth-bound counterparts. Unfortunately, human immune functions are also reduced in space. This unique situation creates a seemingly perfect storm of conditions under which, were a pathogenic species to proliferate, the results could be devastating.
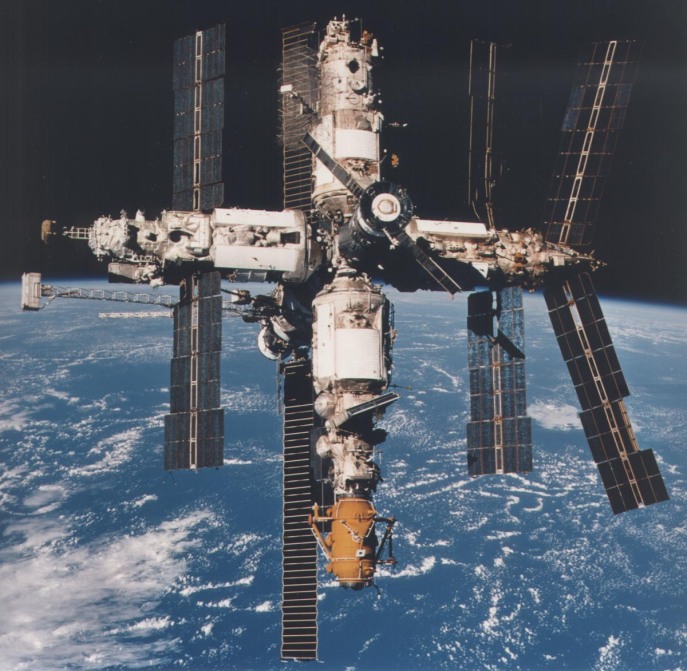
The mir space station, owned and operated by the Soviet Union and then Russia, experienced problems with biofilms for the 15 years it was in orbit
NASA has recognized for years that a better understanding of how biofilms survive in microgravity is important for the success of any long-term manned space missions. But little is known about the development and physiology of biofilm mats in microgravity, making it hard to predict the likelihood of a pathogenic outbreak. To investigate this, scientists cultured Pseudomonas aeruginosa, a common model organism and a human pathogen, on two Space Shuttle Atlantis missions under microgravity conditions. They simultaneously ran ground control cultures at the Kennedy Space Center to compare biofilm growth rates, cell densities and architecture.
The results were startling. The number of viable cells, total biomass, and the thickness of biofilms were all significantly higher on spaceships than on the ground. This result was robust regardless of the carbon source or level of nutrients provided to the bacterial culture.
Depending on the organism and environmental conditions, biofilms can organize themselves in a variety of ways and produce characteristic structures. On Earth, P. aeruginosa biofilms are flat on static surfaces and mushroom-shaped under hydrodynamic conditions (i.e., with water flowing across them). Using confocal laser-scanning microscopy, the researchers compared the structures of P. aeruginosa biofilms grown in spaceflight and normal gravity. While under normal gravity P. aeruginosa formed flat biofilms, in space biofilms exhibited a novel “column and canopy” structure, with cells aggregating in tight-knit columns close to the adhesion surface, overlaid with a dense, canopy-like mat furthest from the surface.
Cellular flagella and pili important in maintaining biofilm structure and surface adhesion under normal gravity. A study recently published in Science even suggests that the appendages known as type IV pili may allow bacteria to stand upright and “walk” during the early stages of biofilm development. To test whether such cell structures are also important in space, the researchers created mutant strains that lacked the genetic capacity to produce flagella or pili. While mutants lacking pili were still able to produce column and canopy biofilms, mutants lacking flagella were not. Thus, flagellar motility appears to play a key role in the formation of unique column and canopy structures in space.
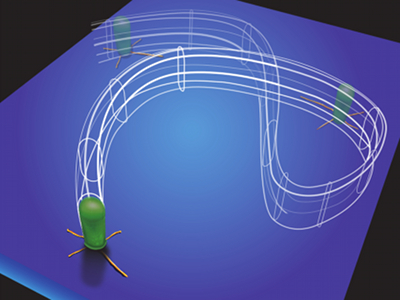
Artistic conception of a bacteria using type IV pili to walk during early biofilm development. Credit: UCLA
This study is the first carefully replicated scientific experiment demonstrating that spaceflight affects the community-level behavior of bacteria. The findings described here carry the profound implication that microbes are capable of adapting to non-terrestrial environments through altered behavioral patterns. More studies are clearly needed to understand the full range of behavioral adaptations exhibited by bacteria in microgravity, but it is clear any future long-term space missions will have to take these tiny organisms- and their potential human health risk- seriously.