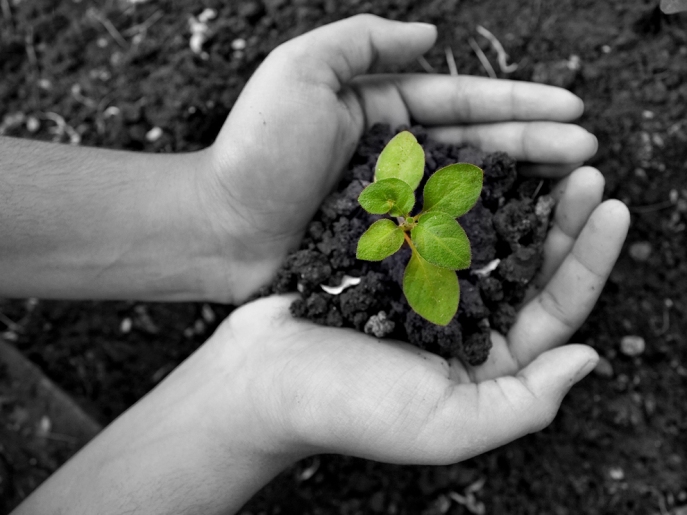
Soil is the most microbially diverse habitat on Earth, and contains twice as much carbon as living plants and the atmosphere combined. Source: National Geographic
When you look at soil, you probably see dirt. When I look at soil, I see billions of microorganisms, crawling atop one another, consuming the dead in a feasting frenzy that stops for nothing save a deep freeze. I see microbes and their enzymes, the digestive juices that break down, transform and release all the energy tied up in our planet’s terrestrial ecosystems.
Through their remarkable ability to decompose nearly anything that comes their way, soil microbes collectively represent a planetary recycling factory, one that takes carbon- the structural unit behind all living matter- from dead organic matter back to the atmosphere as carbon dioxide (CO2). Soils “exhale” CO2 for the same reason you and I exhale CO2. We’ve eaten something, broken down the carbon bonds that hold it together, and extracted all the energy that we could. CO2 is the generic waste product of cellular metabolism, the last bit of carbon that our own metabolic inefficiency precludes us from using. Ecologists have coined the term soil respiration to describe the collective exhale of microbial carbon decomposition.
Breathing matters, but in soil, it’s complicated
This earthly exhale is an event of profound significance to the biosphere. It dictates how much carbon remains in soil, and how much returns to the atmosphere, where acts as a heat-trapping greenhouse gas that helps regulate Earth’s climate. Biogeochemists- scientists who study the planetary cycling of elements, like carbon- are working to understand how microbes contribute to the global exhale of Earth’s soils. What makes them breathe slower? Faster? How does this influence the distribution of carbon across a forest? Across the planet? Over a year? A century? Understanding what makes soil microbes breathe will allow scientists to make better predictions about our planet’s future. How will human disturbances, like climate change, urbanization, or fertilization affect this important carbon pathway?
In order to predict why soils exhale, we need to first understand who or what’s breaking down carbon. And it turns out, when you really get down into the dirt, things get a bit more complicated. You see, microbes don’t actually accomplish all that carbon digestion by themselves. Enzymes- proteins that act as catalysts by facilitating specific reactions- mediate nearly every step in soil carbon decomposition. Some of these enzymes reside inside living microbes, but many are released outside the cell, into the environment. These “extracellular enzymes”, or exoenzymes, act as independent entities from their microbial producers. This means exoenzymes can-and often do- persist in soil long after their microbial parent dies. By chemically associating with soil minerals, exoenzymes can form stable complexes that resist drought, pH changes, even attack and degradation by other enzymes.
And finally, to make matters even more complicated, some carbon decomposition occurs by entirely abiotic (non-living) processes. Certain soil minerals, such as iron oxides, strip electrons form organic matter in a process known as oxidation. Oxidation is important for the breakdown of large, complex molecules such as lignin, the primary building block of wood.
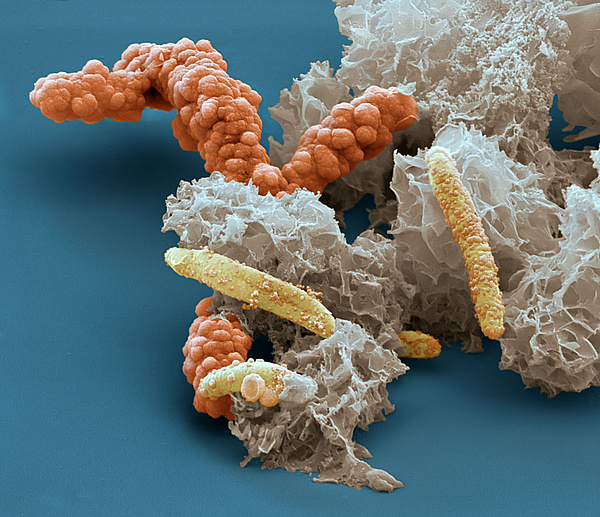
Scanning electron microscope image of iron oxidizing bacteria Acidovorax sp. BoFeN1, encrusted in iron minerals. Microbes, enzymes and minerals are often intimately associated in soils, making it difficult to separate out their contributions to biochemical reactions. Source: Eye of Science, Reutlingen
Exoenzymes and soil microbes represent two different pieces of the decomposition puzzle. Abiotic carbon oxidation represents a third. Since these different aspects of decomposition may respond differently to environmental change, we’d like a way of separately measuring their contributions to the soil carbon cycle. But separating exoenzymes, microbes and minerals, all of which not only coexist within nanometers of each other, but are often chemically bonded, is no easy task. Soil microbial ecologists have long recognized, sometimes acknowledged, but largely ignored our inability to separate the living from the non-living in soil carbon decomposition.
Separating the living and the dead
Finally, we may have a roadmap for solving a problem that has eluded microbial ecologists for years. Last month, a group of researchers led by Dr. Joshua Schimel at the University of California, Santa Barbara, published the first study that rigorously attempts to separate cellular carbon metabolism from exoenzyme activity in soils. The study is currently in press in the journal Soil Biology & Biochemistry.
To separate cellular from extracellular metabolism in soil, the researchers recognized the need for a method that could disable living cells while leaving exoenzymes intact. This is no trivial problem. Heat can be used to sterilize soil, but high heat can also disrupt exoenzyme activity and accelerate the break-down of other organic compounds. Toxic chemicals like sodium azide (NaN3) can inhibit most microbial activity, but a small fraction of microbes develop resistance.
Two potential methods the researchers decided to test are chloroform fumigation and gamma irradiation. Chloroform, a nonpolar and hydrophobic (water-avoiding) molecule, is chemically inert but nonetheless deadly. Chloroform buries itself in the lipid bilayer of cell membranes, causing membranes to become disorganized, and eventually rupture. While some exoenzymes are clearly not affected by chloroform, others may be. Gamma radiation is a powerful, ionizing radiation that punctures cell membranes and causes them to burst. Gamma radiation is frequently used to sterilize food and medical equipment. Very few studies have measured the effect of gamma radiation on exoenzymes. The scientists compared the efficacy of these to techniques to autoclaving, a more traditional sterilization method in which a sample is subjected to very high heat.
Having chosen several sterilization methods, the researchers set up an experiment to test how cellular and extracellular soil carbon metabolism would respond. They collected soils from a California grassland, and sterilized samples using each method. From each sterilized soil, they attempted to culture viable cells. They also added a vital stain (one that differentiates living and dead cells), and used fluorescent microscopy to evaluate the number of living and dead cells.
To evaluate extracellular metabolism, they measured the activity of eight exoenzymes produced by a wide range of soil microorganisms. The eight chosen enzymes degrade common components of soil organic matter (lignin, cellulose, chitin and proteins). They included six hydrolases, enzymes that break specific chemical bonds, and two oxidases, which indiscriminately strip electrons from organic molecules. Hydrolases like beta-glucosidase are important for degrading molecules with predictable, repeating structures, such as cellulose. Oxidases play a central role in breaking down larger, more complex structures, similarly to abiotic oxidation.
To evaluate cellular metabolism, the researchers used a technique called “substrate induced respiration” (SIR). SIR involves adding a small molecule (typically an amino acid or glucose) that cells can metabolize completely, and measuring CO2 loss. The CO2 generated from an SIR experiment indicates the metabolic potential of live cells in a sample.
They found chloroform and gamma irradiation both led to a 1,000 fold reduction in the number of living cells. Autoclaving was a more powerful sterilization technique; reducing the number of live cells approximately 100,000 fold. Most exoenzyme activities were only modestly reduced by chloroform or gamma irradiation. However, sterilization did reduce the activity of two enzymes, alpha-glucosidase and beta- xylosidase by approximately 75%. It’s possible that consistent reductions in activity across all enzymes may have had a proportionally greater effect on these two, which had the lowest activity in non-sterilized soils.
From these initial findings, what can we conclude? Both chloroform and gamma radiation effectively sterilize soils (if we can accept the fact that a few hardy spores might resist these treatments). Both treatments also keep exoenzyme activity intact (keeping in mid low-activity soils will probably experience proportionally larger reductions). A few caveats here and there, but by and large both techniques appear promising.
But what about cellular metabolism? Did sterilization successfully eliminate this, as well? Your gut reaction might be that this is a silly question. If we’re killing all living cells, we must also be killing cellular activity, right? That’s at least what the researchers initially thought.
The SIR results tell a different story. The scientists added a number of different simple carbon substrates, all of which can are broken down during basic cellular metabolic processes like glycolysis and the citric acid cycle, or TCA cycle.Following sterilization, respiration from glucose and amino acid substrates was halted. However, respiration from TCA-cycle substrates, including pyruvate, citrate and ketoglutarate, was reduced but not eliminated. How could dead cells continue respiring? The authors speculate that certain dehydrogenases, the enzymes that break down TCA cycle-substrates, are able continue functioning outside the controlled environment of the cell. This finding was completely unexpected. It calls into question many assumptions about one of the most fundamental energy-harvesting processes in cellular biology, while further blurring the line between microbial metabolism and environmental metabolism.
Another surprise came from the oxidase enzymes. While all hydrolytic enzymes were killed by high heat in the autoclaving treatment, oxidative reactions persisted. The authors speculate the oxidative activity they measured in autoclaved soil is the result of abiotic processes, supporting the notion that a large amount of oxidative decomposition in soils can be caused by mineral catalysis.
Taken as a whole, this study finds both chloroform fumigation and gamma irradiation to be promising methods for separating cellular and extracellular metabolism. Some refinements are certainly needed- finding a way to separate living from non-living oxidation, for instance. But both methods already promise to unravel new mechanisms for microbial carbon metabolism, as highlighted by the finding that some TCA- cycle enzymes can persist after cell death. Now scientists can start using these sterilization treatments to measure the lifetime of exoenzymes in the environment. Right now we can only guess at how long exoenzymes stick around once they’re produced. Understanding the long-term persistence of these enzymes in the environment will help us to better gauge the capacity of soils- even in the absence of living cells- to continue digesting the planet’s carbon.
Blankinship, J., Becerra, C., Schaeffer, S., & Schimel, J. (2014). Separating cellular metabolism from exoenzyme activity in soil organic matter decomposition Soil Biology and Biochemistry, 71, 68-75 DOI: 10.1016/j.soilbio.2014.01.010